Fracture networks are vital for enhanced geothermal systems, as they improve fluid circulation and boost thermal energy extraction. Using techniques like hydraulic or CO2 fracturing, these complex networks allow for better heat transfer and uniform distribution. Advanced simulation approaches, including THMC modeling and finite element methods, help understand the intricate interactions within these networks. By integrating new characterization tools, you can enhance accuracy and effectiveness in geothermal applications. Discover how these developments could shape the future of energy solutions.
Key Takeaways
- Fracture networks are essential for efficient thermal energy extraction in enhanced geothermal systems, enabling effective fluid circulation in low-permeability rocks.
- Complex fracture geometries, including secondary and branched fractures, enhance heat extraction and improve thermal energy transfer rates.
- Advanced modeling approaches, such as thermal-hydraulic-mechanical-chemical (THMC) models, help assess and predict fracture sustainability and performance.
- Integration of new characterization tools, like nanosensors, improves the precision of measurements related to fracture network properties and connectivity.
- Ongoing research into advanced materials and high-temperature experiments is crucial for overcoming challenges in natural systems and enhancing geothermal system deployment.
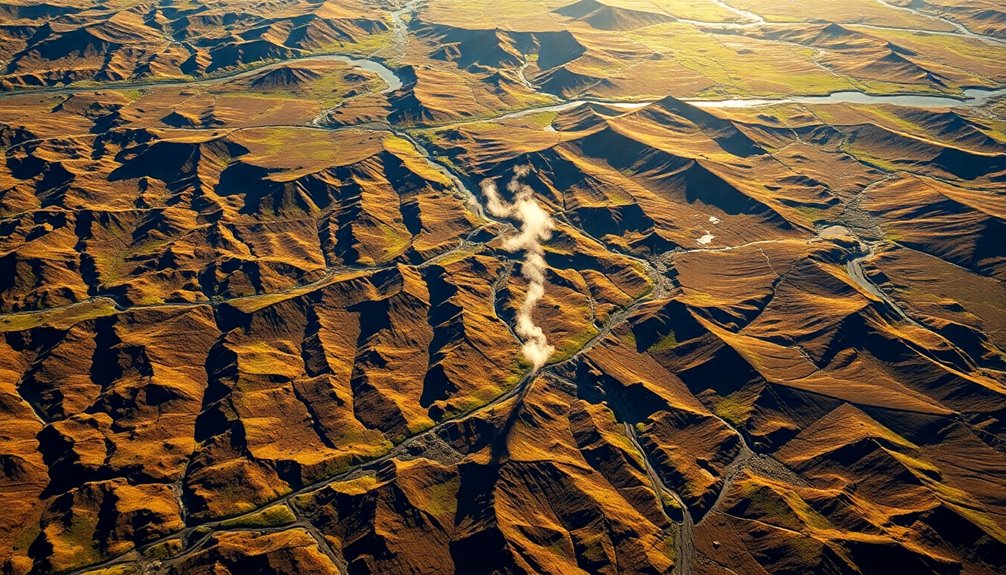
Fracture networks play a crucial role in enhanced geothermal systems (EGS), as they enable the efficient extraction of thermal energy from hot, low-permeability rocks. By providing pathways for fluid circulation, effective fracture networks significantly enhance heat extraction. To create these networks, techniques like hydraulic fracturing and CO2 fracturing are commonly employed. Each method has its strengths; while hydraulic fracturing uses high-pressure fluids to create complex networks, CO2 fracturing requires lower pressures, making it environmentally favorable.
Fracture networks are essential for efficient thermal energy extraction in enhanced geothermal systems, utilizing techniques like hydraulic and CO2 fracturing.
The geometry of these fracture networks is vital. Complex networks, featuring secondary and branched fractures, outperform simple patterns by allowing better heat extraction. When you have closer fracture spacing, thermal energy transfer speeds up, optimizing the overall process. Moreover, the orientation of fractures relative to fluid flow plays a crucial role in thermal breakthrough times, with complex networks generally leading to more uniform heat distribution. The geometry of the fracture network significantly influences thermal breakthrough.
Modeling approaches are key to understanding and improving these systems. Thermal-hydraulic-mechanical-chemical (THMC) models assess fracture sustainability and performance, while numerical simulations help in modeling complex fracture growth and fluid-rock interactions. Though the discrete element method effectively simulates fluid-rock interactions, it struggles with complex networks. The finite element method offers detailed fluid-structure coupling but requires significant computational resources.
Characterization of fracture networks is also evolving. New tools, like nanosensors, allow for precise measurements of pressure, temperature, and fracture aperture. Well-to-well tests provide valuable data on connectivity and performance, integrating findings with computational modeling for accurate network characterization.
However, challenges remain in extrapolating these findings to natural systems, indicating a need for further research. As advanced materials and high-temperature experiments develop, the future of enhanced geothermal systems looks promising, with fracture networks at the forefront of unlocking sustainable energy solutions.
Frequently Asked Questions
How Do Fracture Networks Affect Geothermal Energy Efficiency?
Fracture networks significantly affect geothermal energy efficiency by influencing heat extraction.
If you have wider fracture spacings, you'll reduce interference, enhancing heat flow. Complex networks often distribute heat more evenly, leading to better performance.
However, you must consider fracture conductivity and the number of fractures; too few or overly conductive fractures can hinder efficiency.
Ultimately, optimizing these parameters is crucial for maximizing the geothermal system's output and ensuring effective energy harnessing.
What Types of Fractures Are Most Beneficial for Geothermal Systems?
For geothermal systems, horizontal fractures are most beneficial due to their efficient heat extraction capabilities.
You're looking for higher fracture density, which enhances heat exchange by reducing cooling rates.
Complex networks with branched patterns improve stability and efficiency.
Lower conductivity in some fractures can minimize heat loss, while optimal spacing maximizes the heat extraction area.
How Are Fracture Networks Detected and Analyzed?
Imagine standing on a rugged cliff, where hidden fractures shape the earth beneath you. You can detect these networks using UAV technology for aerial imaging, borehole logging for in-depth analysis, and structural scanlines for surface surveys.
Nanosensors and fluid logging track minute changes within reservoirs. For analysis, you'd employ Discrete Fracture Network models and thermal-hydraulic simulations, revealing how these intricate networks influence fluid flow and heat transfer in geothermal systems.
What Are the Environmental Impacts of Exploiting Fracture Networks?
Exploiting fracture networks can lead to several environmental impacts.
You might notice changes in groundwater quality due to chemical reactions, and excessive water usage could strain local resources.
The infrastructure development often disrupts ecosystems and habitats, affecting biodiversity.
Noise pollution from drilling could disturb wildlife, while visual impacts alter landscapes.
Additionally, improper management of heat and chemical releases can harm local ecosystems.
It's essential to address these issues through effective regulations and sustainable practices.
Can Fracture Networks Change Over Time in Geothermal Reservoirs?
Of course, fracture networks don't change over time—said no geothermal engineer ever!
In reality, these networks are dynamic. They evolve due to factors like hydraulic stimulation, mechanical stress, and fluid injection. You'll notice that high-pressure fluid can enhance connectivity and permeability.
Even natural tectonic processes contribute. So, yes, if you're monitoring a geothermal reservoir, expect the fracture networks to shift and adapt, affecting their efficiency and overall performance.
Conclusion
In conclusion, understanding fracture networks in enhanced geothermal systems is crucial for optimizing energy extraction. Did you know that studies show up to 90% of the geothermal energy in some reservoirs is stored in these intricate networks? By harnessing advanced simulation techniques, you can gain insights into how these fractures influence fluid flow and heat transfer. This knowledge not only boosts efficiency but also paves the way for more sustainable energy solutions. Let's dive deeper into this fascinating field!